Bowen discovered that as magma cools in the laboratory, certain minerals crystallize first. At successively lower temperature, other minerals begin to crystallize as shown in Figure 3. As the crystallization process continues, the composition of the melt liquid portion of a magma, excluding any solid material continually changes.
For example, at the stage when about 50 percent of the magma has solidified, the melt will be greatly depleted in iron, magnesium, and calcium, because these elements are found in the earliest formed minerals. But at the same time, it will be enriched in the elements contained in the later forming minerals, namely sodium and potassium. Further, the silicon content of the melt becomes enriched toward the latter stages of crystallization. Bowen also demonstrated that if a mineral remained in the melt after it had crystallized, it would react with the remaining melt and produce the next mineral in the sequence shown in Figure 3.
For this reason, this arrangement of minerals became known as Bowen's reaction series. On the upper left branch of this reaction series, olivine, the first mineral to form, Ml] react with the remaining melt to become pyroxene. This reaction will continue until the last mineral in the series, biotite mica, is formed.
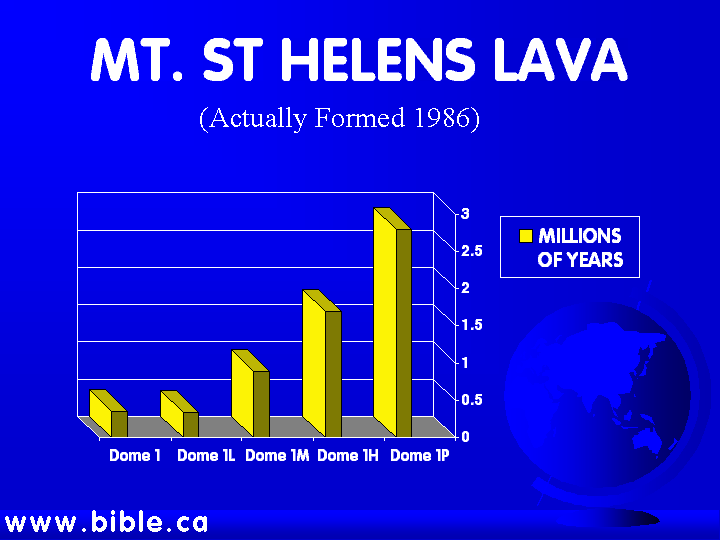
This left branch is called a discontinuous reaction series because each mineral has a different crystalline structure. Recall that olivine is composed of a single tetrahedra and that the other minerals in this sequence are composed of single chains, double chains, and sheet structures, respectively. Ordinarily, these reactions are not complete so that various amounts of each of these minerals may exist at any given time.
The right branch of the reaction series is a continuum in which the earliest formed calcium-rich feldspar crystals react with the sodium ions contained in the melt to become progressively more sodium rich. Oftentimes the rate of cooling occurs rapidly enough to prohibit the complete transformation of calcium-rich feldspar into sodium-rich feldspar. In these instances, the feldspar crystals will have calcium-rich interiors surrounded by zones that are progressively richer in sodium.
During the last stage of crystallization, after most of the magma has solidified, the remaining melt will form the minerals quartz, muscovite mica, and potassium feldspar. Although these minerals crystallize in the order shown, this sequence is not a true reaction series. Bowen demonstrated that minerals crystallize from magma in a systematic fashion. But how does Bowen's reaction series account for the great diversity of igneous rocks?
It appears that at one or more stages in the crystallization process, a separation of the solid and liquid components of a magma frequently occurs. This can happen, for example, if the earlier formed minerals are heavier than the liquid portion and settle to the bottom of the magma chamber as shown in Figure 3. This settling is thought to occur frequently with the dark silicates, such as olivine.
When the remaining melt crystallizes, either in place or in a new location if it migrates out of the chamber, it will form a rock with a chemical composition much different from the original magma Figure 3. In many instances the melt which has migrated from the initial magma chamber will undergo further segregation. As crystallization progresses in the " new" magma, the solid particles may accumulate into rocklike masses surrounded by pockets of the still molten material.
It is very likely that some of this melt will be squeezed from the mixture into the cracks which develop in the surrounding rock. This process will generate an igneous rock of yet another composition. The process involving the segregation of minerals by differential crystallization an separation is called fractional crystallization. At any stage in the crystallization process the melt might be separated from the solid portion of the magma. Consequently, fractional crystallization can produce igneous rocks having a wide range of compositions.
Bowen successfully demonstrated that through fractional crystallization one magma can generate several different igneous rocks. However, more recent work has indicated that this process cannot account for the relative quantities of the various rock types known to exist. Although more than one rock type can be generated from a single magma, apparently other mechanisms also exist to generate magmas of quite varied chemical compositions. We will examine some of these mechanisms at the end of the next chapter. Separation of minerals by fractional crystallization.
UCSB Science Line
Illustration of how the earliest formed minerals can be separated from a magma by settling. The remaining melt could migrate to a number of different locations and, upon further crystallization, generate rocks having a composition much different from the parent magma. Faure discusses fractional crystallization relating to U and Th in his book p.
These values may be taken as an indication of the very low abundance of these elements in the mantle and crust of the Earth. In the course of partial melting and fractional crystallization of magma, U and Th are concentrated in the liquid phase and become incorporated into the more silica-rich products. For that reason, igneous rocks of granitic composition are strongly enriched in U and Th compared to rocks of basaltic or ultramafic composition.
Progressive geochemical differentiation of the upper mantle of the Earth has resulted in the concentration of U and Th into the rocks of the continental crust compared to those of the upper mantle. The concentration of Pb is usually so much higher than U, that a 2- to 3-fold increase of U doesn't change the percent composition much e. Finally, we have a third quotation from Elaine G.
Recent Opinions
Kennedy in Geoscience Reports, Spring , No. Contamination and fractionation issues are frankly acknowledged by the geologic community. If this occurs, initial volcanic eruptions would have a preponderance of daughter products relative to the parent isotopes. Such a distribution would give the appearance of age. As the magma chamber is depleted in daughter products, subsequent lava flows and ash beds would have younger dates. Such a scenario does not answer all of the questions or solve all of the problems that radiometric dating poses for those who believe the Genesis account of Creation and the Flood.
It does suggest at least one aspect of the problem that could be researched more thoroughly. So we have two kinds of processes taking place. There are those processes taking place when lava solidifies and various minerals crystallize out at different times.
There are also processes taking place within a magma chamber that can cause differences in the composition of the magma from the top to the bottom of the chamber, since one might expect the temperature at the top to be cooler. Both kinds of processes can influence radiometric dates. In addition, the magma chamber would be expected to be cooler all around its borders, both at the top and the bottom as well as in the horizontal extremities, and these effects must also be taken into account. For example, heavier substances will tend to sink to the bottom of a magma chamber.
Also, substances with a higher melting point will tend to crystallize out at the top of a magma chamber and fall, since it will be cooler at the top. These substances will then fall to the lower portion of the magma chamber, where it is hotter, and remelt. This will make the composition of the magma different at the top and bottom of the chamber. This could influence radiometric dates.
- blind dating cast.
- im dating a mamas boy true life.
- Radiometric dating - Wikipedia;
- dating your roommates sister.
- dating site cyprus.
- How reliable is geologic dating?;
This mechanism was suggested by Jon Covey and others. The solubility of various substances in the magma also could be a function of temperature, and have an influence on the composition of the magma at the top and bottom of the magma chamber. Finally, minerals that crystallize at the top of the chamber and fall may tend to incorporate other substances, and so these other substances will also tend to have a change in concentration from the top to the bottom of the magma chamber.
There are quite a number of mechanisms in operation in a magma chamber. I count at least three so far -- sorting by density, sorting by melting point, and sorting by how easily something is incorporated into minerals that form at the top of a magma chamber. Then you have to remember that sometimes one has repeated melting and solidification, introducing more complications.
Radiometric Dating is Accurate
There is also a fourth mechanism -- differences in solubilities. How anyone can keep track of this all is a mystery to me, especially with the difficulties encountered in exploring magma chambers. These will be definite factors that will change relative concentrations of parent and daughter isotopes in some way, and call into question the reliability of radiometric dating. In fact, I think this is a very telling argument against radiometric dating.
Another possibility to keep in mind is that lead becomes gaseous at low temperatures, and would be gaseous in magma if it were not for the extreme pressures deep in the earth. It also becomes very mobile when hot. These processes could influence the distribution of lead in magma chambers. Let me suggest how these processes could influence uranium-lead and thorium-lead dates: The following is a quote from The Earth: The magnesium and iron rich minerals come from the mantle subducted oceanic plates , while granite comes from continental sediments crustal rock.
The mantle part solidifies first, and is rich in magnesium, iron, and calcium. So it is reasonable to expect that initially, the magma is rich in iron, magnesium, and calcium and poor in uranium, thorium, sodium, and potassium. Later on the magma is poor in iron, magnesium, and calcium and rich in uranium, thorium, sodium, and potassium. It doesn't say which class lead is in. But lead is a metal, and to me it looks more likely that lead would concentrate along with the iron. If this is so, the magma would initially be poor in thorium and uranium and rich in lead, and as it cooled it would become rich in thorium and uranium and poor in lead.
Thus its radiometric age would tend to decrease rapidly with time, and lava emitted later would tend to look younger. Another point is that of time. Suppose that the uranium does come to the top by whatever reason. Perhaps magma that is uranium rich tends to be lighter than other magma. Or maybe the uranium poor rocks crystallize out first and the remaining magma is enriched in uranium.
Would this cause trouble for our explanation? It depends how fast it happened. Some information from the book Uranium Geochemistry, Mineralogy, Geology provided by Jon Covey gives us evidence that fractionation processes are making radiometric dates much, much too old. The half life of U is 4.
Thus radium is decaying 3 million times as fast as U At equilibrium, which should be attained in , years for this decay series, we should expect to have 3 million times as much U as radium to equalize the amount of daughter produced. Cortini says geologists discovered that ten times more Ra than the equilibrium value was present in rocks from Vesuvius. They found similar excess radium at Mount St.
Helens, Vulcanello, and Lipari and other volcanic sites. The only place where radioactive equilibrium of the U series exists in zero age lavas is in Hawiian rocks. We need to consider the implications of this for radiometric dating.